This is not a theological issue: when marine researchers talk about the deepest depths of the oceans, they call them the “adale” zone, named after the […]
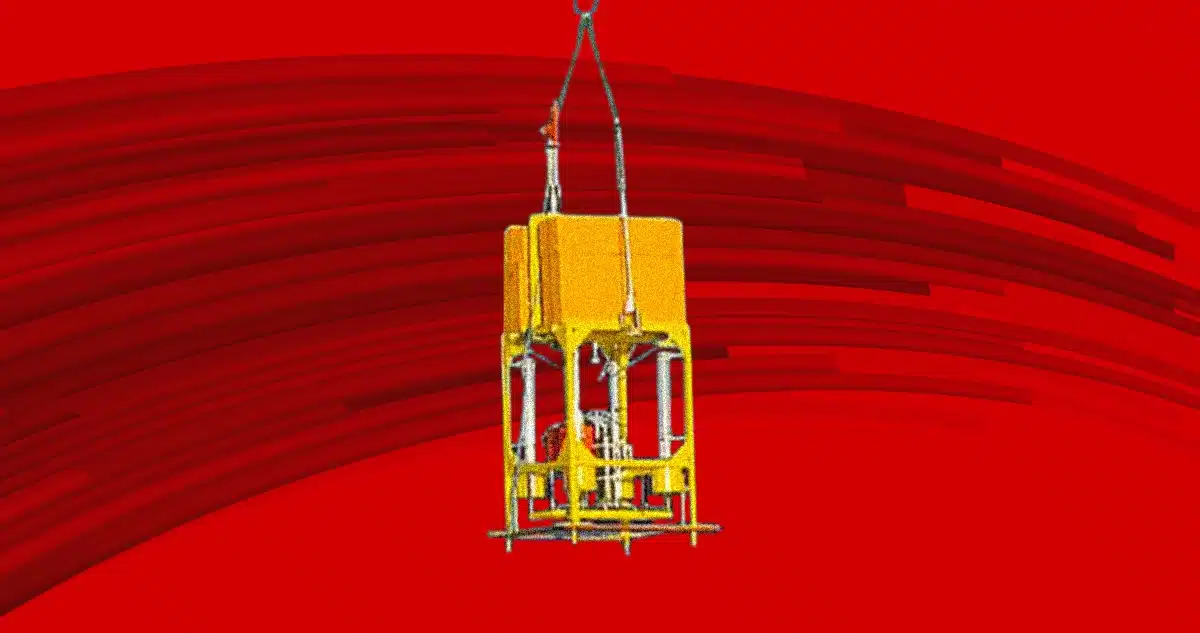
This is not a theological issue: when marine researchers talk about the deepest depths of the oceans, they call them the “adale” zone, named after the Shadow Realm used by the ancient Greeks.
However, they assume much more life is there than in mythological Hades.
The pits of the deep sea may even impact the world’s climate, and micromotors are helping to find answers.
Mars is millions of kilometers away from Earth. However, its surface is better studied than the bottom of ocean trenches, which are only eight to eleven kilometers away from the sea surface. The biological and chemical processes that occur are, in fact, still largely unknown. A research project, not coincidentally called “Hades-ERC,” is trying to change this by providing completely new information about the depths of the oceans. The pioneer has been Professor Ronnie Glud of the University of Southern Denmark in Odense.
“In marine biology, there is a simple rule of thumb,” he says. “The deeper you go to the bottom, the less life you find there.” For as depth increases, so does cold and darkness. Only a fraction of the food produced in the water near the surface reaches great depths. In addition, water pressure increases by 1 bar every ten meters. At a depth of 10,000 meters, the pressure of about 1,000 bar is a thousand times greater than what we find, for example, on the shores of Sardinia. “But gravity manages to exert its action even in this environment. Some organic matter that reaches the ocean floor eventually settles in ocean pits, where it accumulates.”
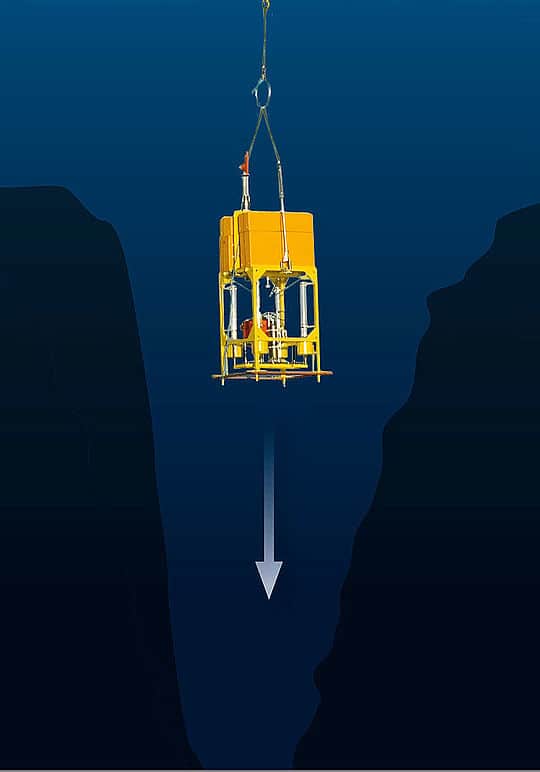
A reservoir for organic matter
That is why, in 2013, Professor Glud was not surprised to find highly active microbial communities at a depth of almost eleven kilometers. At that time, he had his instrumentation submerged in the Mariana Trench of the Western Pacific. “We found more organic matter at depths below 10,000 meters than at 6,000 meters,” the marine researcher explains. “Therefore, we assume that the pits have a very important influence on our seas’ carbon/nitrogen balance. Although responsible for only 2 percent of the ocean area, they could have a major effect on carbon occurrence and, more generally, on climate events.”
The “Hades-ERC” project aims to go deep (literally) into the matter to enable a better understanding of the processes occurring in pits. The European Research Council, part of the European Union, funds it. These are advanced grants called “Advanced Grants” that amount to 2.5 million euros and allow scientists to conduct long-term, open-ended basic research. In addition to Odense’s Glud department, the project involves marine biologists from the University of Copenhagen and other marine research institutes in Germany, Japan, and Scotland. The sophisticated instrumentation was developed by a collaboration between the Odense team and a German team led by Dr. Frank Wenzhoefer and stationed at the Max Planck Institute in Bremen.
The project is scheduled to last five years, and studies will be conducted, starting in the fall of this year, in three Pacific trenches: the Japan Trench, the Atacama Trench, and the Kermadec Trench, at depths between 8,100 and 10,900 meters. These formations were selected for the difference in organic loading in the waters above. In this sense, they offer their microbial inhabitants widely varied conditions.
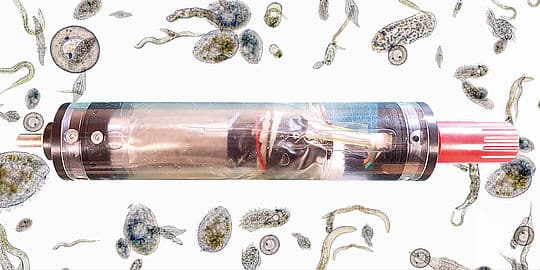
Robots instead of submarines
Although there have already been manned dives at these depths, submarines would not be the most appropriate choice for conducting in-depth research on seafloor sediments. Therefore, the team involved in this project has developed robots that autonomously descend to the seafloor and perform preprogrammed studies. They are equipped with sensors that, among other things, can measure the oxygen uptake of bacteria — a value from which the amounts of organic matter processed can be deduced.
Other sensors help find answers to the question of whether microbes in the deep sea breathe oxygen, nitrate, or sulfate. “To survive the extreme conditions of the deep sea, the bacteria in question must be very different from their peers in shallower waters,” asserts Professor Glud. “For example, their membranes and enzymes must function completely differently. The ‘how,’ specifically, is precisely what we are trying to find out.”
Wanting to study microbes themselves is a very special challenge. Having adapted to an environment of incredible water pressure, it is impossible to bring them trivially to the surface. In fact, during ascent, they would turn into a kind of “soup,” as the Danish researcher metaphorically describes. That is why the “Hades-ERC” project robots are equipped with tools that can inject a fixing agent into the sediment that can keep the microorganisms intact during their recovery.
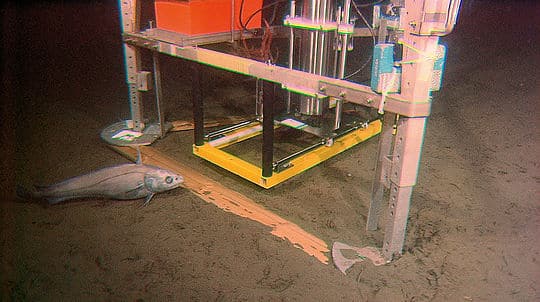
The pre-requisite: pressure resistance
Suppose microbes are to be protected from the pressure that decreases during surface ascent. In that case, special precautions must also be taken to protect the instruments installed in the robots from the extreme pressure of ocean pits. For this reason, both sensors and sediment handling tools are properly designed for this environment and thus able to withstand such pressure. In any case, to do their job, they must be able to come into contact with the sediment and subsequently be moved to different positions. Responsible for this movement are DC micromotors, complete with planetary gearboxes and encoders.
While some components are housed in a pressure-resistant titanium cylinder, some devices, such as motors and gearboxes, can only do their work when they come in contact with the surroundings to be studied. “Therefore, we housed these components in another cylinder inside a small flexible membrane filled with an inert liquid,” Professor Glud explains. “The membrane ensures that the water pressure acts on the enclosed components without differential pressure arising. Because that would cause the motors to collapse.”